
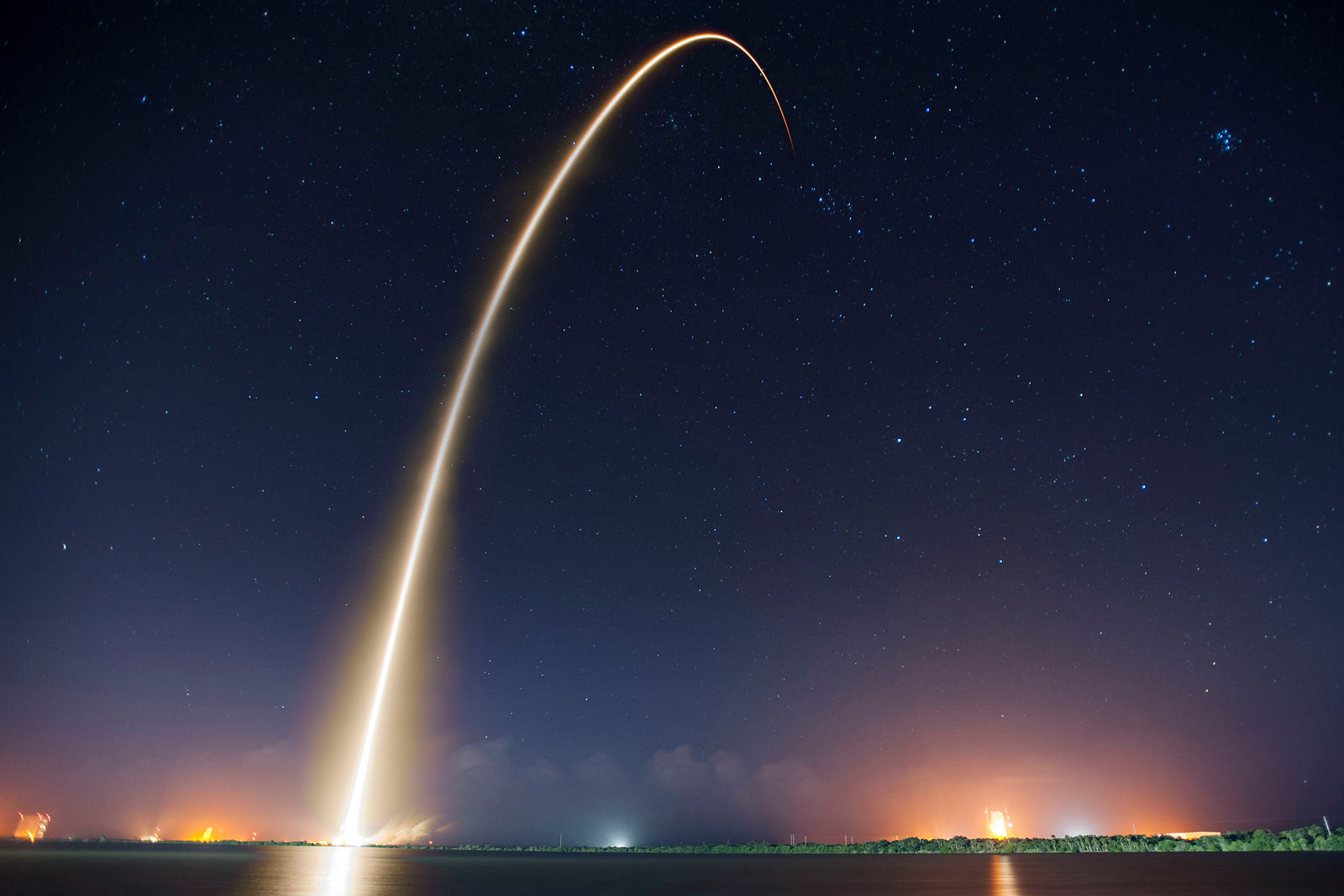
They are also subject to their own operational limitations. These methods have been shown to provide data as reliable as conventional methods ( Costa et al., 2006 Alimenti et al., 2020), but they may be costly to deploy, therefore limiting their widespread adoption of use. In recognition of these challenges, the development of non-contact sensors for velocity measurements (e.g., surface velocity radar), and non-intrusive approaches for acquiring bathymetric data (e.g., bathymetric LiDAR, radar, photogrammetry) is now facilitating the non-contact and autonomous monitoring of fluvial flows ( Costa et al., 2006 Flener et al., 2013 Javernick et al., 2014 Alimenti et al., 2020). Whilst this technology offers some advantages over traditional gauging techniques (e.g., ultrasonic sensors, electromagnetic current meters), they fail to overcome a critical limitation in that they require contact with the water-body, which may not be viable during high-flow conditions or where physical access to the channel is not possible. This has led to advances in the ability to reliably collect data over large areas, most importantly, entire water columns ( Neill and Hashemi, 2018). Of the recent advances in sensing river flows, arguably the most pronounced is the widespread adoption of acoustic Doppler current profiler (aDcp) technologies ( Kostaschuk et al., 2005). Further understanding in areas that are lacking, such as environmental challenges, is vital if image velocimetry is to be used as a method for the extraction of river flow information across the range of hydro-geomorphic conditions.Īdvances in understanding of hydrological processes and the characterisation of river flows have been driven by development of novel sensing instruments, data acquisition platforms, and new analytical techniques (e.g., Chen et al., 2007 Assem et al., 2017 Mishra et al., 2019). For example, the role of external environmental conditions on the performance of image velocimetry being a key aspect that is currently lacking research. However, there are some stages in the process that are not conclusive with one set method and require user intuition or further research.
#Optimage piv how to
For most sub-systems, there is clear research or precedence indicating how to best perform analysis. We present literature from a range of sources from across the hydrology and remote sensing literature to suggest circumstances in which specific approaches are best applied. The focus of each section is to provide examples from the wider literature for best practice, or where this is not possible, to provide an overview of the theoretical basis and provide examples to use as precedence and inform decision making. The image velocimetry work-flow is compartmentalised into sub-systems of: capture optimisation, pre-processing, processing, and post-processing. This research collates, synthesises, and presents current understanding related to the application of particle image velocimetry (PIV) and particle tracking velocimetry (PTV) approaches in a fluvial setting. As such, for those new to image velocimetry, it may be hard to decipher which methods are suited for particular challenges. This has resulted in the development of a multitude of techniques, with varying practice being employed between groups, and authorities.
#Optimage piv software
Research within this topic has largely focussed on developing specific aspects of the image velocimetry work-flow, or alternatively, testing specific tools or software using case studies. Large-scale image velocimetry is a novel approach for non-contact remote sensing of flow in rivers.
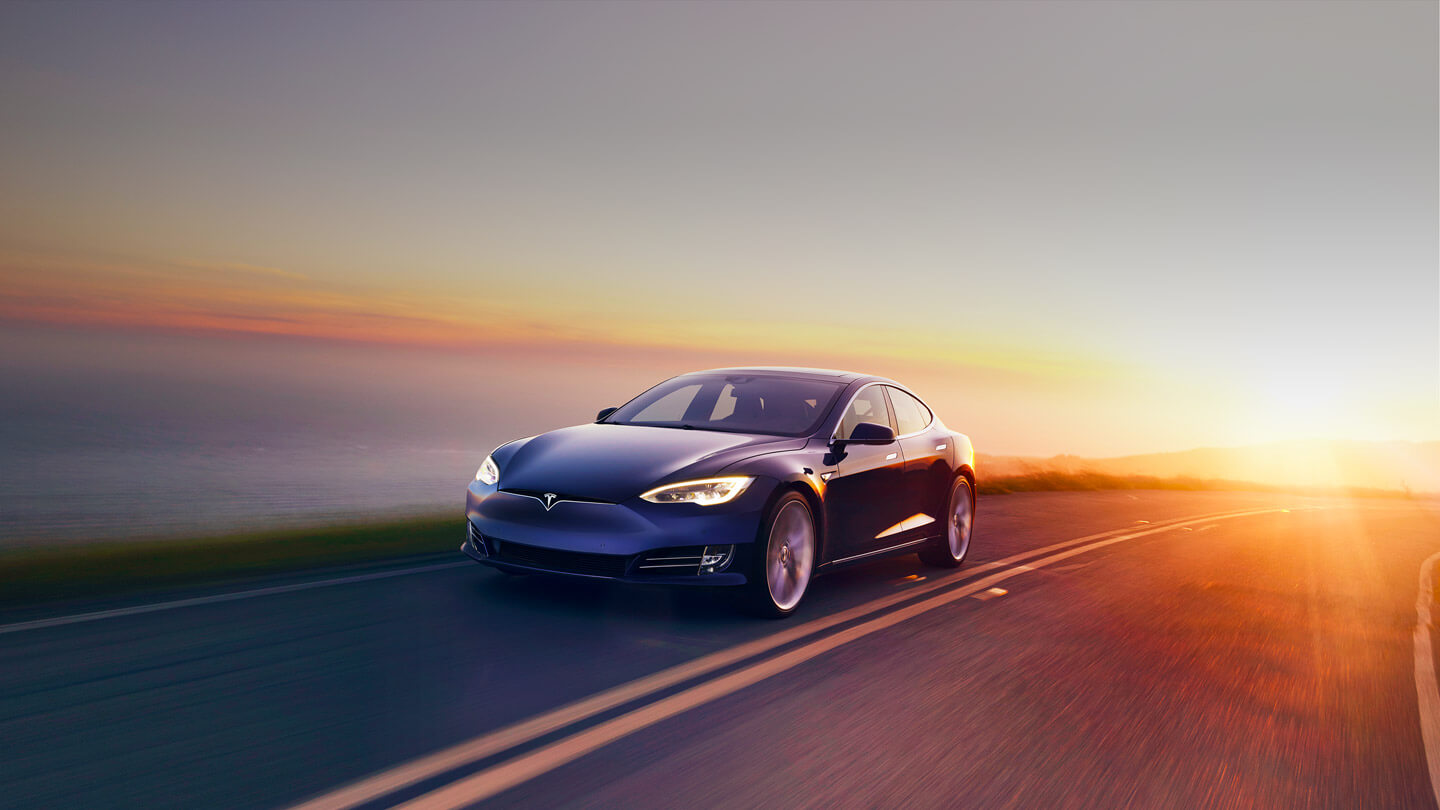
2School of Engineering, Newcastle University, Newcastle upon Tyne, United Kingdom.
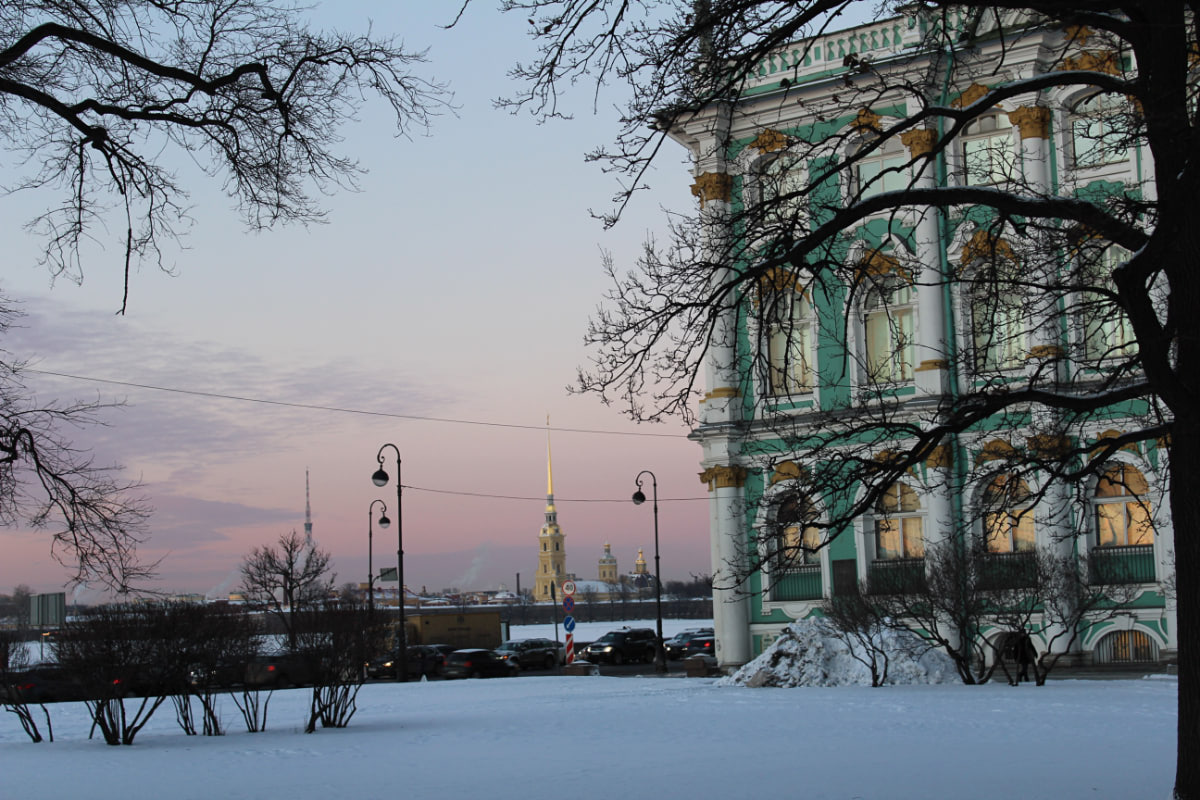
1School of Geography, Politics and Sociology, Newcastle University, Newcastle upon Tyne, United Kingdom.
